Green Shipping: How Technology is Reshaping the Trillion-Dollar Maritime Industry
- Chandrama Prasad Vishawakarma
- Nov 8, 2023
- 10 min read
Updated: 5 days ago
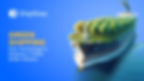
In the ever-shifting landscape of maritime trade ties, the unrelenting issue of emissions weighs heavy on the tides. The colossal maritime industry is a substantial contributor to global emissions, accounting for 3% of global levels. Figuratively, if the entire industry was considered as a country, it would be the 7th largest Greenhouse gas(GHG) contributor in the world! A wake-up call resonates across the shipping sector in an increasingly carbon-conscious world, urging a shift towards greener horizons.
The emission statistics cast a stark shadow over the industry's current state. The call has spurred a revolution in maritime technology. Ingenious minds in ship design and engineering are embarking on a quest to weave sustainability into the very fabric of shipping. Green technologies once considered the province of dreams, now take the form of reality. From wind-assist propulsion to hydrogen fuel cells, the maritime landscape is morphing into a crucible of cleaner possibilities.
Governments and international bodies are enacting stringent regulations to steer this transformation. The International Maritime Organization's (IMO) Sulphur cap mandates cleaner fuels, pushing shipowners to embrace low-Sulphur alternatives or install exhaust scrubbers. Stringent Energy Efficiency Existing Ship Index (EEXI) norms set the course for existing ships to improve efficiency or face operational limitations.
In the crucible of greener technology, the future of shipping takes shape. Each innovation and regulation is a chisel striking at the mold of an industry in metamorphosis. The waves that bear the vessels of tomorrow carry the whispers of change, as the maritime realm navigates towards a more sustainable and responsible horizon.
As the industry recognizes its role as both disruptor and custodian, the story of Green Shipping is one of hope – where technology plays the role of both the enabler and the hero, steering us towards a future where progress and preservation coexist harmoniously.
1. Need for Green Technologies in Shipping:
The International Maritime Organization (IMO)'s cornerstone maritime legislation, MARPOL Annex VI, places strict restrictions on ship emissions. This framework sets the predicament for decreasing sulfur oxide (SOx) and nitrogen oxide (NOx) emissions to protect ecosystems and reduce air pollution. The most recent significant accomplishment in this process is IMO 2020, a rule that enforces a global sulfur cap of 0.5% on marine fuels and significantly lessens the reliance on heavy fuel oil (HFO) and its related sulfur emissions.
HFO is currently the target of this regulatory transition owing to its infamously bad effects on both air and water quality. Controlling sulfur emissions is essential for protecting human health and the health of delicate marine ecosystems.
Shipowners and operators can comply with these laws using green technologies without incurring fines or being subject to limitations imposed by member states. The use of green technology is required to achieve current sustainability targets stated set by nations, especially in European/US waters.
By the year 2050, the International Maritime Organization wants to cut shipping emissions by at least 50%, and it is practically only possible to reach this number with the aid of green technology. Imperatively, this is the need of the hour.
2. Short-Term Solutions:
While diving into the realm of changes the industry has to undergo in the foreseeable future, it is clear that shipping is a capital-intensive project. It takes billions in investment to redraw changes in a traditional industry been driven by internal combustion engines for decades, and as we embrace this monumental change, the solutions can be clubbed under short-term solutions and long-term ones.
Exhaust Gas Cleaning Systems (EGCS):
EGCS, widely recognized as scrubbers, serve as a pivotal technology in the reduction of sulfur emissions within maritime operations. Scrubbers are ingeniously incorporated into ships to mitigate sulfur oxides (SOx) found in exhaust gases produced during the combustion of heavy fuel oil (HFO). This sophisticated system employs diverse techniques, such as employing seawater or alkaline solutions, to cleanse exhaust gases. This proactive measure neutralizes sulfur content before its release into the atmosphere, ensuring compliance with sulfur emissions regulations whilst enabling the continued use of HFO.
EGCS benefits are manifold. Firstly, it enables vessels to persistently harness existing HFO infrastructure persistently, circumventing the need for an immediate shift to low-sulfur fuels. This pragmatic approach is economically sound, particularly during the transitional phase towards cleaner energy sources. Furthermore, scrubbers showcase the exceptional potential for elevated sulfur emissions reduction compared to a singular reliance on low-sulfur fuels. Impressively, scrubbers achieve sulfur removal efficiencies surpassing 90%, thereby substantially mitigating the ecological footprint of maritime activities.
2. Very Low Sulphur Fuel Oil (VLSFO):
The ascent of Very Low Sulphur Fuel Oil (VLSFO) as a viable alternative to traditional high sulfur fuel oil (HFO) has been noteworthy. This marine fuel variant boasts a significantly curtailed sulfur content, consistently below 0.5% sulfur by weight, in full compliance with the International Maritime Organization's stringent sulfur cap regulation.
VLSFO garners distinction through multiple merits. Firstly, it directly tackles the conundrum of sulfur emissions. By embracing VLSFO over HFO, ships promptly adhere to sulfur regulations, manifesting reduced emissions of sulfur oxides (SOx) and consequent amelioration of air quality. Secondly, VLSFO substantiates its ecological credentials by minimizing the sulfur and pollutant content vis-à-vis HFO. This translates into a tangible reduction in greenhouse gas emissions, amplifying the trajectory towards environmental equilibrium.
3. Ballast Water Treatment Systems (BWTS):
In parallel, the maritime realm grapples with another ecological quandary—the propagation of invasive species via ballast water. Ballast water, crucial for vessel stability, often houses a mélange of organisms including bacteria, microbes, minute invertebrates, and potentially invasive species' eggs or larvae. Discharging this water in foreign ecosystems instigates ecological imbalances and jeopardizes biodiversity.
To address this challenge, Ballast Water Treatment Systems (BWTS) emerge as the harbinger of ecological equilibrium. These systems deftly cleanse ballast water, systematically removing or neutralizing the existing organisms. The array of technologies employed spans filtration, ultraviolet irradiation, and chemical treatment, each ensuring the eradication or inactivation of organisms.
The deployment of BWTS signifies a resolute stride towards curbing invasive species proliferation, thereby conserving marine ecosystems. By subjecting ballast water to pre-discharge treatment, ships assume responsibility for minimizing the ecological footprint, in tandem with observance of international regulations like the Ballast Water Management Convention instituted by the International Maritime Organization. This convention, with its exacting treatment standards, seeks to abate the environmental risks linked to the transfer of harmful organisms via ballast water.
3. Long-Term Solutions:
These include changes that will define the future of global trade and the way goods get transported across the seven oceans. As mentioned before, it would take a few hundred billion dollars to embrace change in this space which constitutes the long-term plans laid out by a culmination of public-private partnerships.
Waste heat recovery systems: They utilize the heat generated during ship operations. Ships produce amounts of heat from different sources, such, as engines, exhaust gases and cooling systems. By using waste heat recovery systems we can convert this heat into useful energy, like electricity or thermal energy for various onboard processes. Its benefits include a marked reduction in fuel usage and improved energy efficiency.
Waste-to-energy systems allow us to convert waste materials produced on ships into energy. Waste Energy is then converted into heat, electricity, or even biofuels using incinerators, gasifiers, or anaerobic digestion. Utilizing waste as a resource waste to energy systems contributes to waste management practices while also generating energy. This technology assists in reducing the amount of waste generated onboard ships minimizing disposal at sea and providing an energy source for ship operations.
The implementation of waste heat recovery and waste-to-energy systems, in shipping offers benefits. By enhancing energy efficiency and reducing fuel consumption, these technologies contribute to sustainability.
Moreover, they play a role, in enhancing waste management practices thereby minimizing the effects of waste disposal on marine ecosystems. Furthermore, these technologies can optimize energy usage, resulting in increased efficiency and reduced operating expenses, ultimately enhancing the competitiveness of shipping companies.
In implementing these technologies, it is important to take into account factors such as limited space availability, technical feasibility, and regulatory considerations.
No Ballast ships: The concept of "no ballast" ships, which's a pioneering approach, to designing vessels, is gaining popularity due to its potential in addressing the risks associated with ballast water. Traditionally used for stability and balance, ballast water has become a source of introducing species and ecological dangers. No ballast ships offer a solution by implementing measures to ensure stability, such as onboard water treatment systems, movable weights and inventive hull designs. This eliminates the risks associated with ballast water while promoting conservation and protecting biodiversity.
2. Air Lubrication: This introduces a layer of compressed air bubbles between the ship's hull and the water surface. This significantly reduces resistance. A study conducted by the Marine Research Institute Netherlands (MARIN) suggests that air lubrication systems could potentially decrease fuel consumption by up to 15% for container ships resulting in improvements, in overall fuel efficiency.
3. Nozzle fins: Also known as speed nozzles, they play a role in improving the efficiency of propulsion. Their main function is to redirect water flow, around the propellers reducing turbulence and maximizing thrust while minimizing energy wastage. Research indicates that the implementation of speed nozzles can lead to propulsion efficiency improvements ranging from 5% to 15%.
As an example, a case study conducted on a bulk carrier vessel demonstrated that the use of speed nozzles resulted in a 10% decrease in fuel consumption, during testing.
Improved hull paints: To counteract the fuel inefficiency caused by biofouling, modern hull paints known as antifouling coatings have been developed. These coatings prevent organisms like barnacles and algae from attaching to the hull, which reduces drag and consequently leads to fuel consumption. Advanced formulations like silicone-based coatings have demonstrated fuel consumption reductions of up to 10% highlighting their impact on operational efficiency.
Alternative modes of propulsion:
The future of global trade is green, whether companies accept it or not. To reduce GHG emissions, the world's future fleet will have to rely on a broader range of fuels, propulsion solutions, and energy efficiency measures. For example, the use of liquefied natural gas (LNG) as a fuel can reduce CO2 emissions by up to 25%, while biofuels can reduce CO2 emissions by up to 90%. Let’s explore those options, and see which ones are the most viable, both economically and scalability-wise:
a) Liquefied Natural Gas (LNG): LNG is a clear, colorless, and non-toxic liquid that is formed when natural gas is cooled to -162°C at atmospheric pressure. The cooling process reduces the volume of natural gas by a factor of 600, making it easier and more cost-effective to transport and store.
The main component of LNG is methane (CH4), the hydrocarbon fuel with the lowest carbon content and therefore with the highest potential to reduce CO2 emissions (maximum reduction: roughly 26 per cent compared to HFO). The use of LNG as a fuel can reduce CO2 emissions by up to 25%, compared to conventional petroleum-based ship fuels.
In addition to reducing CO2 emissions, the use of LNG also eliminates emissions of sulfur oxides (SOx) and particulate matter (PM). Engines fueled by LNG still reduce NOx by 40% without exhaust gas treatment and particle emissions are reduced by 95% and more. Because LNG does not contain sulfur, these emissions are eliminated.
LNG can be used in existing gas engines without requiring any modifications. Liquefied biogas or methane produced from hydrogen and carbon in a power-to-fuel process can also be used in LNG fuel systems and gas engines without requiring any modifications. None of these liquefied methane-based gases causes any tank-to-propeller carbon dioxide emissions (TTP emissions), provided that carbon from renewable sources is used.
In conclusion, LNG is a promising alternative fuel for the maritime industry due to its potential to reduce greenhouse gas emissions and eliminate harmful emissions such as SOx and PM. However, decision-makers must keep in mind that other low and zero-emission sources of liquid methane can be used as drop-in fuels, and the most cost-competitive option may vary depending on the ship type and trade.
b) Methanol: Methanol (CH3OH), a liquid fuel possessing the lowest carbon composition and the highest hydrogen concentration among liquid fuels, stands out. Methanol can be derived from various feedstock resources, predominantly natural gas or coal. Renewable sources such as black liquor from pulp and paper mills, agricultural waste, forest thinning, and even CO2 captured from power plants also serve as avenues for methanol production.
In terms of heating value, methanol falls below conventional petroleum-based fuels, leading to methanol fuel tanks needing approximately 2.5 times more space than oil tanks for comparable energy content. With a flashpoint of 11°C to 12°C, methanol is categorized as a low-flashpoint fuel. Moreover, it can be transformed into dimethyl ether (DME), serving as a viable fuel for diesel engines.
The cost of methanol per unit of energy content typically aligns with natural gas prices, often reflecting a higher value concerning energy content. Methanol's cost per unit of energy content ranged between European heavy fuel oil (HFO) and marine gas oil (MGO) prices between 2010 and 2013. Subsequently, there has been a marginal increase in methanol prices, currently reinstating levels observed in 2013. Notably, the decrease in oil prices has positioned methanol as a pricier option compared to distillate marine fuels.
c) Hydrogen: It is a colorless, odorless, and non-toxic gas. Hydrogen has the highest energy content per unit of weight of any fuel, and when used in fuel cells, it produces only water and heat as byproducts. It can be produced from various sources, including natural gas, biomass, and renewable electricity through electrolysis.
For use on ships, hydrogen can either be stored as a cryogenic liquid, as compressed gas, or chemically bound. The energy density per mass of hydrogen is approximately three times the energy density of heavy fuel oil (HFO), but the volumetric density of liquefied hydrogen (LH2) is only 7% of that of HFO. This results in approximately five times the volume compared to the same energy stored in the form of HFO. When stored as a compressed gas, its volume is roughly 10 to 15 times the volume of the same amount of energy when stored as HFO.
While hydrogen has the potential to reduce greenhouse gas emissions, it is important to consider its production and transportation methods, as well as its potential for leakage during use.
d) Biofuels: These act as energy carriers derived from the conversion of primary biomass or biomass remnants into liquid or gaseous fuels. Among the most promising biofuels for maritime applications are hydro-treated vegetable oil (HVO), fatty acid methyl ester (FAME), and liquefied biogas (LBG), although choices exist as well.
The integration of biofuels stems primarily from the objective of curbing greenhouse gas (GHG) emissions. Various studies emphasize sustainable biofuels as a pivotal avenue for deep-sea shipping to fulfil the International Maritime Organization's (IMO) target of reducing GHG emissions by a minimum of 50% by 2050, juxtaposed against 2008 levels. The extent of GHG mitigation significantly hinges on factors such as feedstock and the production process.
Additional facets of biofuel creation harbor controversies, including concerns related to land use and socio-economic implications. Multiple standards and initiatives aim to address these concerns. Biofuels stemming from advanced procedures that leverage sustainable feedstocks can yield considerable GHG reductions while mitigating potential ancillary effects.
As maritime pioneers, we stand at a crossroads of progress and preservation. Green Shipping's narrative brims with hope, where technology assumes the twin mantles of enabler and hero, steering the sector toward an era where environmental equilibrium coexists with innovation. The maritime industry’s evolution unveils an alliance between progress and sustainability, promising a voyage toward a more conscientious, cleaner horizon.
Written by ANKUR KUNDU